Abstract
Students' misconceptions concerning colour phenomena and the apparent complexity of the underlying concepts—due to the different domains of knowledge involved—make its teaching very difficult. We have developed and tested a teaching device, the addition table of colours (ATC), that encompasses additive and subtractive mixtures in a single conceptual model and presents colour as a perceptual response to light. The ATC was used as the focus of an introduction to colour phenomena in a middle class setting, showing significant educational gains among the students.
Export citation and abstract BibTeX RIS
1. Introduction
The world around us is full of colour, so students possess a system of beliefs and intuitions derived from their personal everyday experiences, leading to the construction of a common sense theory which is often incompatible with scientific laws. Non-scientific information and pervasive ideas are frequent in many physics topics and have been identified and analysed in order to determine their origin and to find ways to overcome them.
A substantial body of research has revealed many naive conceptions about colour phenomena, concluding that students' ideas of colour are the result of their experiences with gouaches, watercolours and coloured pencils; for the majority of students, 'adding' lights is the same as painting [1, 2]. Moreover, many commercially available colour mixing devices, such as colour paddles or the traditional colour wheel, are not supported by physical principles [2].
Furthermore, some studies have indicated that flaws in students' understanding of light propagation [3–7] are responsible, at least in part, for their own understanding of colour phenomena [1]. It seems that, in spite of Newton's correct scientific explanations in the 17th century on this subject, children continue to express very similar ideas to those defended two thousand years earlier by Aristotle, considering darkness as an ingredient of colour [1] and colour as a property of the object.
The most frequent ideas students have about colour phenomena are collected and organized in table 1 [1, 2, 8, 9]. The majority of these ideas were obtained from interviews where students were encouraged to use diagrams to demonstrate their own ideas, accompanied by their verbal explanations. These in-depth assessments of the participants' pre-instruction conceptions of colour phenomena revealed that the naive conceptions are not randomly organized in students' minds; instead they appear through coherent internal schemes, used consistently in different contexts, namely through mental models.
Table 1. Childrens' conceptions regarding colour phenomena.
Colour is a property of the object and is independent of the light falling on it or any filters placed over it |
When white light passes through a coloured filter, the filter adds colour to the light |
The colour of an object is a property of the object which remains unchanged under white light but could be changed by coloured light |
Dark colours cover light colours |
Mixtures of light follow the same rules as mixtures of paints |
The colour of an object is a mixture of its own colour and the colour of the incident light |
Light has colour and gives its own colour to the object |
White light is colourless and clear, enabling you to see the real colour of an object |
Black and white are colours |
The colour of a shadow is always the colour of the light source |
An additional complication for early teaching of colour phenomena lies in its combination of physical, psychological and physiological aspects.
In a simplified first approach, leaving many psychological and physiological details aside, colour is related to the spectral characteristics of electromagnetic radiation as it reaches the retina. There are three types of photoreceptors (cones) present in the retina, having different sensitivities to the electromagnetic spectrum, often called red, green and blue, according to the wavelength to which they have maximum sensitivity. Today, we regard the Young–Helmholtz three-receptor theory as the first step in explaining colour perception. In spite of its limitations, this model is largely accepted when it comes to teaching this subject at a introductory level because it explains the main physical features of the process.
The colour of an object and the resulting colour of mixtures of paints or of lights are different aspects of the same phenomena—and should be accounted for by a single theoretical framework. However, students introduce several independent misleading explanations of these three processes, which teaching practice often fails to take into consideration—or, even worse, reinforces. An example is the classical circular representations of additive and subtractive mixtures, quite common in schoolbooks, using essentially identical representations for substantially different operations [7, 8]. Since traditional science instruction on the subject of colour has often unsuccessfully relied heavily on textbook-based approaches, this is a serious problem that can only be overcome if teachers possess a solid body of scientific and pedagogical knowledge.
Previous research has established that the teaching of colour requires a theoretically effective instructional strategy, including a detailed comparative analysis and synthesis of additive and subtractive mixtures [7, 8]. Thus, the development of a theoretical understanding of this subject is essential to appropriate learning. Students need to understand additive and subtractive mixtures as belonging to a single theoretical framework able to explain the colour of objects and mixtures of lights or paints.
2. ATC model
We propose a simple mental model, the addition table of colours (ATC), to introduce colour phenomena. This model, inspired by the concept of colour perception as the outcome of the collective response of the three types of visual cones, presents additive and subtractive mixtures in a single theoretical framework that accounts for colour perception as the result of the interaction of the physical properties of light with the physiological and psychological features of the human visual system.
As previously mentioned, the retina contains thousands of cones (cells sensitive to visible radiation), which can be classified, in a simplistic way, into three types, depending on the type of radiation to which they are most sensitive. When light activates only one of these three types of cones we have the perception of 'green', 'blue' or 'red', depending on the type of stimulated cone. This is the reason why these colours are called primary. The immense variety of colours we perceive is no more than a combination of these three colours—in other words, the result of different activity in the three types of sensitive cells. If the retina had a detection system with five components, we would have five primary colours, and colour perception would result from the overlap of five signals.
We can thus consider the perceived colour as defined in a three-dimensional space of red, green and blue, (R, G, B), as, in fact, is the case of the RGB model of colour in many computer systems. The simplified ATC model we used for instruction reduces the possible values of R, G and B to either 0 (absence) or 1 (presence), with the exception of the discussion of grey. As we proceed with the demonstrations, it becomes clear to the students that they are dealing with a rather restricted palette of colours. If this issue is raised it offers a good opportunity to explain that red, green and blue can be present with different intensities, not just present or absent.
Students are given an addition table, such as shown in figure 1, which shows how to add light; that is, how the presence of two primary colours in light is perceived. In addition to the 'equations' of figure 1, students are also taught that black is the absence of any light (R=0, G=0, B=0), and white light contains equal amounts of red, green and blue light (R=1, G=1, B=1), that is, an equal intensity of stimulation of the three types of cones; by changing the amount of light, in the same proportion, we get the shades of grey between black and white.
Figure 1. The ATC and additive mixtures.
Download figure:
Standard image High-resolution imageThese simple concepts and figure 1 provide tools that allow students to discover and explore colour phenomena. To generate colours other than those in this model, we would have to consider continuous, independent, variations of the intensities of green, red and blue.
Common objects or filters do not emit light by themselves (they are not light sources); they reflect, and can also absorb a fraction of the light that illuminates them. We are, thereby, required to use the 'minus' (−) sign to indicate that light has been absorbed (subtracted). Therefore, a cyan filter (one that looks cyan under white light) absorbs red (− RED) and a green filter absorbs blue and red (− BLUE − RED).
Broadly speaking, the ATC is based on a single representation with which students build simple equations for colour combinations by attributing the plus sign (+) when they have light sources, and the minus sign (−) in the case of filters or light absorbing objects. Only the primary colours (red, blue and green) are used in all terms of the equations, just as in the case of the human optical system.
Figure 2. The ATC and subtractive mixtures.
Download figure:
Standard image High-resolution imageNote that students can derive the relations given in figure 2 from the ones in figure 1. The classical triads of circles, once a source of misunderstanding, can now be built entirely by students, giving a fundamental reasoning tool. This intellectual activity allows students to understand that the primary colours in the additive mixture are the secondary colours in the subtractive mixture and vice versa. It is instructive to see how we can unite these two types of mixture in one equation and solve the problem of coloured objects illuminated by different coloured lights. Here are two examples.
- (a)What does a green object (under sunlight) look like when covered with a cyan filter and illuminated by yellow incident light?
- (b)What does a magenta object (under sunlight), covered with a green filter and illuminated by white light, look like?
Figure 3 shows an excerpt from a student answer, in a lab worksheet where he/she was asked to explain, with the ATC model, the colour perceived when different types of cellophane (red, green and blue) are placed on yellow plasticine.
Figure 3. Excerpt from a student answer.
Download figure:
Standard image High-resolution imageThe ATC is a scientific tool that takes into account the specific difficulties students have with regard to the comprehension of colour, because it clarifies, unequivocally, how the information carried by light is treated by visual system. The students, creating and solving these equations, become aware of the composition of the incident light and understand how it interacts with matter, because the composition of the transmitted/reflected light is also given by the equation. The result is the response of the visual system. The ATC model, though hardly doing justice to the subtlety and complexity of colour perception phenomena, nevertheless does capture its essential ingredients in manner that is adequate for teaching at this age level. With the ATC model, the concept of colour is simplified, without sacrificing its scientific validity, thereby reducing any perception of arbitrariness that more superficial teaching can produce in students' minds.
3. Implementation
We addressed the teaching of colour, using the ATC model, with 8th grade students (aged 12–13), who undertake, at this age, their first formal introduction to the subject of colour. A total of 454 students were involved in this study; 204 from a control group and 250 from an experimental group. The ATC instruction was part of an interactive colour teaching module developed to use two lectures and one lab class (a total of 180 min). The intervention involved three essential ingredients: (a) lesson plans with well-defined objectives; (b) experimental activities; and (c) teacher training.
To evaluate the effectiveness of the ATC and the intervention programme, a method was devised to assess the students' knowledge before and after instruction. The knowledge assessment questionnaire, simultaneously pre- and post-test, was administered individually to the students from both groups before and after instruction on colour and had three functions.
- (a)To confirm conceptions and ways of reasoning about colour phenomena (diagnostic test).
- (b)To identify the knowledge background of both groups and assess whether the samples are equivalent.
- (c)To evaluate the intervention programme; as it was administered before and after the instruction, it also evaluated the general effectiveness of the instruction in modifying the students' previous common sense conceptions.
In the design of this instrument, the students' misconceptions, already identified by previous researchers, were carefully selected and taken into account when preparing the questions. In the test (multiple choice with the exception of one question), alternative answers reflected the most common and significant misconceptions.
To ensure that questions included in the test did not favour the ATC instruction over the conventional teaching method, some teachers, not involved in the project, were asked to check if such questions adequately met the main objectives of the curriculum guidelines. The validation of the pre- and post-test results was made in two ways. A panel of physics teachers reviewed the first version of the test: two from high school and three from higher education institutions. A pilot test was implemented with 28 students of the 8th grade, before and after teaching on colour phenomena, to determine if everyone understood the questions and agreed with the correct answers. An English translation of the colour test is in the supplementary material (available at stacks.iop.org/PhysED/49/61/mmedia).
4. Results
During the training session, the complexity of colour phenomena required clarification for nearly all the teachers. For instance, none of the eight teachers involved in the project could clearly explain why black, white and grey are not considered colours. In spite of their long experience (most teachers had more than 15 years of teaching), it appeared that they had become aware of a new understanding of the concept of colour and were subsequently sensitive to a new teaching approach.
Teachers unanimously considered this pedagogical practice more effective than their previous one. The ATC model was considered a very simple and efficient way to understand the differences between additive and subtractive mixtures. According to the teachers consulted, the equations form a good exercise in analytical thinking and make colour phenomena easier to understand.
The results of the pre-tests corroborate previous studies regarding typical misconceptions. The frequency of answers to each question are very similar for both groups. The results of the post-test, in both groups, showed an increased score over the pre-test, with a clearly much greater improvement in the experimental group (figure 4). The mean of the post-test results for the experimental group (M = 5.33, SD = 1.554) were considerably higher than for the control group (M = 3.30, SD = 1.766).
It is customary to measure the gain in student knowledge as a result of instruction as G = R2 − R1, where R1(2) is the average percentage score in pre(post)-test; the average normalized gain is given as g = G/(100 − R1), since 100 − R1 is the maximum possible gain. The normalized gain of the experimental group was 64.8% against 24.2% for the control group.
We also analysed, individually, all the questions presented in the test. The experimental group had better results in all questions. In the control group, 11.3% of the students had a positive rating in the pre-test, which rose to 42.6% in the post-test. In the experimental group, the rise was higher, from 15.2% to 86.4%.
Figure 4. Results between schools in both groups before and after the traditional versus ATC instruction.
Download figure:
Standard image High-resolution image5. Discussion and conclusions
A finer statistical analysis shows that the differences between the groups before the colour instruction (ATC versus traditional) are random. More importantly, the pre-tests show that students are unaware of the main principles of colour phenomena, with misconceptions firmly in place before any formal teaching of the subject.
Both groups improved in their conceptual understanding of colour as a result of instruction, yet the gain in knowledge was clearly superior in the group using the ATC. The favourable statistical results were corroborated by the opinion of the teachers involved in the project.
Many components of pedagogical intervention may have affected the result observed, such as, for instance, the motivation of the teachers involved in the project, or the existence of a laboratory class. One cannot claim that the ATC model, by itself, is responsible for the success of the programme, even though the teaching practice was, in fact, built around this model. Separating these various effects (the model, teacher and student motivation, experimental stations, teacher training) is quite difficult in general, due to their obvious interdependence; but that was not the purpose of this investigation, which was rather to verify the effectiveness of this mental model and its proposed implementation as a whole.
This study aims to encourage teachers to implement innovative and straightforward teaching strategies and to close the gap between teaching practice and research in physics education. For this reason, constant interaction between teachers and researchers was important for successive reviews of the material and improvement in the quality of this intervention programme.
Acknowledgments
This work was supported by the European Union, programme POCI 2010 through project Ciência Viva PVI/252.
Biographies
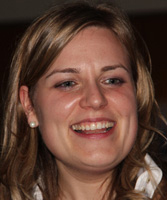
Ana Rita Lopes Mota is a physics and chemistry teacher in middle and high schools. She obtained her PhD in physics education from the Department of Physics and Astronomy at the University of Porto, Portugal, where she remains as a physics education researcher.
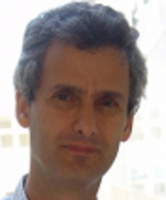
João Lopes dos Santos obtained his PhD at Imperial College, UK, and is a professor of physics at the Department of Physics and Astronomy at the University of Porto. His research is mainly on condensed matter physics theory, and more recently on graphene. He has also coordinated several projects related to physics teaching at middle and secondary levels.